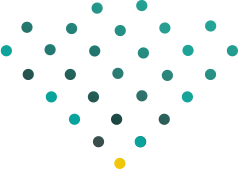
Real Time Monitoring of Helicopter Turbine Blade Temperature Using Passive Wireless Sensor
By Sreekala Suseela, Taofeek Orekan, Swadipta Roy, Joshua McConkey, Reamonn Soto Sensatek Propulsion Technology, Inc. Daytona Beach, USA {spapali, torekan, sroy, josh, rsoto}@rsoto
Abstract— Real time passive wireless temperature sensing system used to monitor helicopter turbine blade temperatures is presented here. The turbine blades were rotating at full speeds (16,000 rpm, equivalent g-load 45,000g) and temperatures above 850°C was monitored and experimentally evaluated and demonstrated. The system uses passive wireless sensor attached to the blades using robust mechanisms and the blade temperature was monitored and recorded in real time. The sensors were interrogated using high temperature silicon dioxide co-axial cable slot antenna using radio frequency resonance-based sensing. The sensor-antenna set up was employed for temperature monitoring up to 1100 °C in high-vibrating metallic environment. Real time temperature monitoring was provided by indigenous software (Turbotrack).
Keywords—passive wireless temperature sensor, co-axial cable slot antenna I.
INTRODUCTION:
The importance of monitoring physical parameters in real time cannot be overstated in modern gas turbine engine design and operation. These parameters, including temperature, pressure, strain, among others, play crucial roles in achieving higher efficiencies and reducing pollution [1]. One of the most critical physical parameters to monitor is the temperature of the turbine blades, as it directly affects the lifespan of these critical components. Overheating of turbine blades can lead to catastrophic failures that not only result in costly repairs and replacements but also endanger the lives of crew and passengers.
To increase the efficiency and lifespan of gas turbine engines, it is vital to detect the temperature profile of the rotating blades in real time. However, monitoring temperatures in harsh environments, such as the interior of a gas turbine where temperatures can exceed 1000°C, poses significant challenges. Traditional methods of temperature sensing using wired sensors are invasive and can cause damage to the blades. Therefore, there is a pressing need for a non-intrusive and robust sensing system that can monitor the temperature of rotating turbine blades accurately and continuously.
Passive wireless sensing technology has emerged as a promising solution to this problem. This technology is capable of sensing in environments that were previously out of reach, including the interior of gas turbines where harsh operating conditions can damage wired sensors. Passive wireless sensors are designed to operate without an external power source, making them suitable for continuous and long-term monitoring of temperatures in harsh environments [2]-[4].
One specific area where the need for passive wireless sensing technology is particularly acute is in the monitoring of helicopter turbine blades. Helicopters rely on gas turbine engines to generate the power required for lift and propulsion. The blades of these turbines operate at high speeds, generating enormous heat that needs to be managed effectively to avoid catastrophic failures. In recent years, there have been several instances of helicopter crashes due to turbine blade failures, emphasizing the critical importance of accurate temperature monitoring. For example, in 2018, a helicopter crashed in New York City, killing five passengers. The National Transportation Safety Board (NTSB) determined that the cause of the accident was a fractured main rotor blade. The blade failure was caused by metal fatigue, which was not detected during the pre-flight inspection [5].
In this paper, we have presented a novel approach for real time monitoring of helicopter turbine blade temperature using passive wireless sensors. The proposed system utilizes RF sensors that are attached to the blades and are wirelessly interrogated by an antenna. The response of the antenna is recorded in real-time, and a time versus temperature graph is plotted, allowing for continuous monitoring of the blade temperature. The system is capable of collecting and saving the temperature data, enabling operators to perform predictive maintenance of the turbine blade, and ensuring the reliability and safety of the helicopter.
Overall, our system presents significant advancement in the field of real-time monitoring of helicopter turbine blade temperature using passive wireless sensors. The system’s nonintrusive, cost-effective, and reliable approach to temperature monitoring has the potential to revolutionize the aviation industry by improving the efficiency, performance, and safety of helicopter operations. We believe that our system’s development represents a significant contribution to the field and provides a foundation for further research and development of real-time monitoring systems for harsh environments.
II. WIRELESS TEMPERATURE MEASUREMENT SETUP
The wireless high temperature measurement set-up can be divided mainly into three parts: Passive wireless sensing, Interrogation antenna and Data collection and recording. These three sections are explained in detail in the sections below:
A.Passive wireless Sensor
The passive wireless sensor is a crucial component of the wireless high temperature measurement set-up, as it is responsible for measuring the temperature of the helicopter turbine blade. In our system, we use a slot antenna integrated with a cavity resonator as an RF passive wireless sensor, as described in [6]. These sensors are fabricated on polymerderived ceramics (PDCs), which have the advantage of high temperature survivability, excellent oxidation/corrosion resistance properties, and flexible manufacturing capabilities.
The sensors work on the principle that the dielectric constant or relative permittivity of the PDC changes with the temperature of the environment in which it is located. When the temperature of the blade with the mounted sensor increases, the resonant frequency reduces, as the dielectric constant of the ceramic substrate material increases over temperature. Fig. 1 shows the dielectric constant derived from the frequency response for commonly used PDC materials at various temperatures.

The slope for the dielectric change for selected materials increases linearly with temperature and as such establishes suitability for our application . To ensure the longevity and reliability of the sensors, commercial platinum paste has been chosen for the metallization of ceramic substrate as platinum has a high melting point of 1768°C and is chemically inert.
The length and width of the slot antenna integrated on the resonator are optimized using CST Microwave Studio software to ensure optimal performance. The sensors are tack-welded onto the turbine blades for blade temperature monitoring, ensuring that they are securely attached to the blade and can withstand the harsh operating conditions of the helicopter turbine.

Fig. 2 shows a fabricated RF passive temperature sensor tack-welded on a turbine blade, illustrating the practical application of the sensor. The sensor was fabricated on Alumina substrate with a thickness of 0.635 mm and the metallization layer used was Platinum. The use of passive wireless sensors in our system eliminates the need for electrical power or wiring, reducing the complexity and cost of the system. Additionally, the non-intrusive sensing mechanism minimizes the risk of damage to the blade, increasing the accuracy and reliability of the temperature measurements. The use of PDCs and platinum metallization ensures that the sensors are reliable, durable, and can withstand the harsh operating conditions of the helicopter turbine. By optimizing the length and width of the slot antenna integrated on the resonator, we can ensure optimal performance of the sensor, providing accurate and reliable temperature measurements.
B. Interrogation antenna
The interrogation antenna is a critical component of the wireless high temperature measurement set-up, as it is responsible for receiving the signal from the passive wireless sensor and extracting the temperature data. In our system, the interrogation antenna works by sending out a wideband signal, which is received by the sensor. The antenna receives the reflected signal from the sensor based on the resonant frequency of the sensor. As the temperature of the blade with the attached sensor increases, the sensor’s resonant frequency changes, and this change in resonant frequency is captured by the interrogation antenna. The shift in resonant frequency with temperature is then used to extract the temperature of the blade material.
The interrogation antenna used in our system requires a wide bandwidth to identify the sensor frequency, which is dependent on temperature. It must also be able to operate at extreme temperatures to withstand the harsh operating conditions of the helicopter turbine. To meet these requirements, we designed a semi-rigid coaxial slot antenna using a semi-rigid, silicon dioxide coaxial cable obtained from Times Microwave System. To ensure that the antenna can operate reliably without damaging the inner conductor of the coaxial cable, slots were introduced along the outer body of the cable. We performed parametric analysis in CST by varying the number of slots, the length of each slot, and the gap between the slots, and the optimized design was chosen for fabrication. To protect the inner conductor of the co-axial cable from high temperature, a layer of platinum paste was used to cover the exposed copper inner conductor of the cable. Fig. 3 shows the two-dimensional view of co-axial slot antenna.

To excite the coaxial slot antenna, we used an R-140, 1-port Vector Network Analyzer (VNA) from Copper Mountain Technologies. The antenna was connected to the VNA, and its S11 response was plotted using the RVNA software. Fig.4 shows the S11 of the coaxial slot antenna measured in free space, indicating a wide bandwidth with an S11 value less than -10 dB from 3.5-14 GHz. To isolate the sensor response from reflections 2023 IEEE Wireless and Microwave Technology Conference (WAMICON) 153 of the antenna, time domain gating was performed. The time domain S11 responses of the antenna with and without the slot sensor is compared in Fig. 5(a). For the case without the sensor, S11 quickly drops to the noise floor. When the sensor is present, there is a significant increase in S11 after the main peak due to the slowly decaying signal at the resonant frequency of the sensor. For a sensing distance of 10 mm, the gating start time is set as 4 ns, and the gating stop time is set to 33 ns where the two curves intersect with each other, as shown in Fig. 5(a). Fig. 5(b) shows the S11 of the antenna in the frequency domain after time domain gating.


The resonant frequency of the sensor can be clearly identified, and in our system, the sensor resonant frequency captured by the interrogating antenna was found to be 4.428 GHz.
The interrogation antenna is a critical component of our wireless high-temperature measurement set-up, providing a reliable and accurate way of extracting temperature data from the passive wireless sensor. The design of the semi-rigid coaxial slot antenna ensures that the antenna can operate reliably at high speeds and withstand the extreme temperatures of the helicopter turbine. By using time domain gating to isolate the sensor response from the reflections of the antenna, we can ensure accurate and reliable temperature measurements, providing critical data for the continuous monitoring of the helicopter turbine blade.
C.Data collection and recording
In the presented system, we use our in-house developed Turbotrack software to collect and record the frequency and temperature data in real time, providing a detailed view of the temperature profile of the helicopter turbine blade.
To ensure that the system is reliable and accurate, we tested it in the lab by simulating high-temperature conditions using a blowtorch. Fig.6 shows the simulated experimental set-up, where the sensor tack-welded on a turbine blade is heated up to 900°C. As the temperature of the blade increases, the resonant frequency shifts towards the left, demonstrating a linear relationship between frequency and temperature. To test the accuracy and reliability of our system, we conducted a ramp-up test of the temperature up to 800°C, as shown in Fig. 7, which illustrates the variation of sensor frequency with temperature.


Our Turbotrack software collects and records the temperature data in real time, providing a detailed view of the temperature profile of the helicopter turbine blade. The data collected by the Turbotrack software is stored in an Excel file, enabling us to analyze the data at a later time for predictive maintenance of the turbine blade.
Turbotrack software ensures temperature accuracy, hysteresis, and sensitivity, making it the most accurate and continuous monitoring technology applied directly to rotating parts. Fig. 8 shows a representative screenshot of the Turbotrack software measuring the real-time temperature with the sensor mounted on a blade while the blade is heated to temperatures greater than 1000°C with a heat gun and gradual cooling down. The data collection and recording component of our wireless high temperature measurement system is critical, enabling us to collect and store accurate temperature data in real time for predictive maintenance of the helicopter turbine blade. By collecting and analyzing the temperature data in real time, we can identify any anomalies or potential failures, enabling us to take corrective action before the turbine blade fails, reducing maintenance costs and increasing the safety of the helicopter.

III. MEASUREMENT RESULTS
The effectiveness of the wireless high-temperature measurement system was evaluated by monitoring the temperature of helicopter turbine blades at full speeds (16,000 rpm, g-load 45,000g) and temperatures above 850°C using sensors developed by Sensatek.

The sensors were tested on a T53 helicopter turbine using multiple sensors with different bonding techniques, including turbine industry standard and proprietary Sensatek in-house techniques. Fig. 9 shows the test environment, where the sensor is tack-welded onto a helicopter engine turbine blade for health monitoring. Antennas were installed and positioned appropriately using tack-welding techniques to ensure accurate and reliable temperature measurements.
The real-time sensor frequency was collected using Turbotrack software, providing us with a detailed view of the temperature profile of the helicopter turbine blade during operation. The data collected by the Turbotrack software enabled us to identify any anomalies or potential failures, enabling us to take corrective action before the turbine blade fails. The results of our testing demonstrated the effectiveness of our wireless high-temperature measurement system in monitoring the temperature of helicopter turbine blades at full speeds and temperatures above 850°C. The use of sensors developed by Sensatek, along with industry standard and proprietary bonding techniques, ensured accurate and reliable temperature measurements, providing critical data for the continuous monitoring of the helicopter turbine blade.
IV. CONCLUSION
In this paper, we have presented a wireless high temperature measurement system for monitoring the temperature of helicopter turbine blades using Sensatek’s passive wireless sensing technology. Our system employs a slot antenna integrated with a cavity resonator as an RF passive wireless sensor to measure the temperature of the environment in which it is located. The system uses a semi-rigid co-axial slot antenna modified to operate as the interrogation antenna for the sensor, with Turbotrack software for real-time data collection and analysis. We conducted tests on the T53 turbine engine, running the system for significantly longer times and installing multiple sensors for precision monitoring. Our experiments demonstrated the effectiveness of our wireless high-temperature measurement system, with Sensatek sensors performing well and accurately reflecting blade metal temperatures.
ACKNOWLEDGMENT
This work is supported by the U.S. Air Force under AFWERX STTR Award Number: FA864920P1012
REFERENCES
[1] M. P. Boyce, Gas Turbine Engineering Handbook, 3rd ed. Amsterdam, The Netherlands: Gulf, 2006, ch. 19.
[2] Carlos R. Mejias-Morillo, Jayaprakash B. Shivakumar, Seng Loong Yu, Blake Roberts, Pedro Cortes, Eric MacDonald, Anton V. Polotai, Eduardo A. Rojas-Nastrucci, “High-Temperature Additively Manufactured C-Band Antennas Using Material Jetting of Zirconia and Micro-Dispending of Platinum Paste”, IEEE Open Journal of Antennas and Propagation, vol.3, pp.1289-1301, 2022.
[3] K. Abudu, U. Igie, O. Minervino, and R. Hamilton, “Gas turbine efficiency and ramp rate improvement through compressed air injection,” vol. 235, no. 4, pp. 866–884, Jun. 2020.
[4] N. Hasan, J. N. Rai, and B. B. Arora, “Optimization of CCGT power plant and performance analysis using MATLAB/Simulink with actual operational data,” Springerplus, vol. 3, no. 1, pp. 1–9, 2014, doi: 10.1186/2193-1801-3-275.
[5] National Transportation Safety Board. (2019). Aviation accident final report: Loss of control and subsequent impact with terrain, Liberty Helicopters, Inc., Eurocopter AS350 B2, N350LH, New York, New York, March 11, 2018 (NTSB/AAR-19/01).
[6] H.T. Cheng, S. Ebadi, and X. Gong, “A low-profile wireless passive temperature sensor using resonator/antenna integration up to 1000˚C,” IEEE AWPL, vol. 11, pp. 369-372, 2012